Originally published on fastradius.com on February 23, 2022
Computer Numerical Control (CNC) machining is a modern, efficient, and automated subtractive manufacturing process that allows manufacturers to make repeatable parts of consistent quality from a wide range of materials, including plastics, metals, and composites. Today, CNC-machined parts can be found in a variety of different industries, from aerospace to automotive to medical.
The beginnings of CNC technologies can be traced back to a few different points in history. The first modern machining tools appeared in 1775 when industrialists developed a boring tool that allowed manufacturers to produce steam engine cylinders with more consistent degrees of accuracy and precision.
The technological forebears of modern computing appeared earlier in the century, however, emerging from the textiles industry. In 1725, Basile Bouchon began using a system of punched holes in paper tape to encode data. Decades later in 1805, Joseph Marie Jacquard refined the process, swapping out the paper tape for sturdy punch cards. When tied in a sequence, the punch cards directed how the fabric was added to the loom, simplifying the process and providing one of the first examples of programming. This machine, the Jacquard loom, is in a way the forebear of our modern computing and automation solutions.
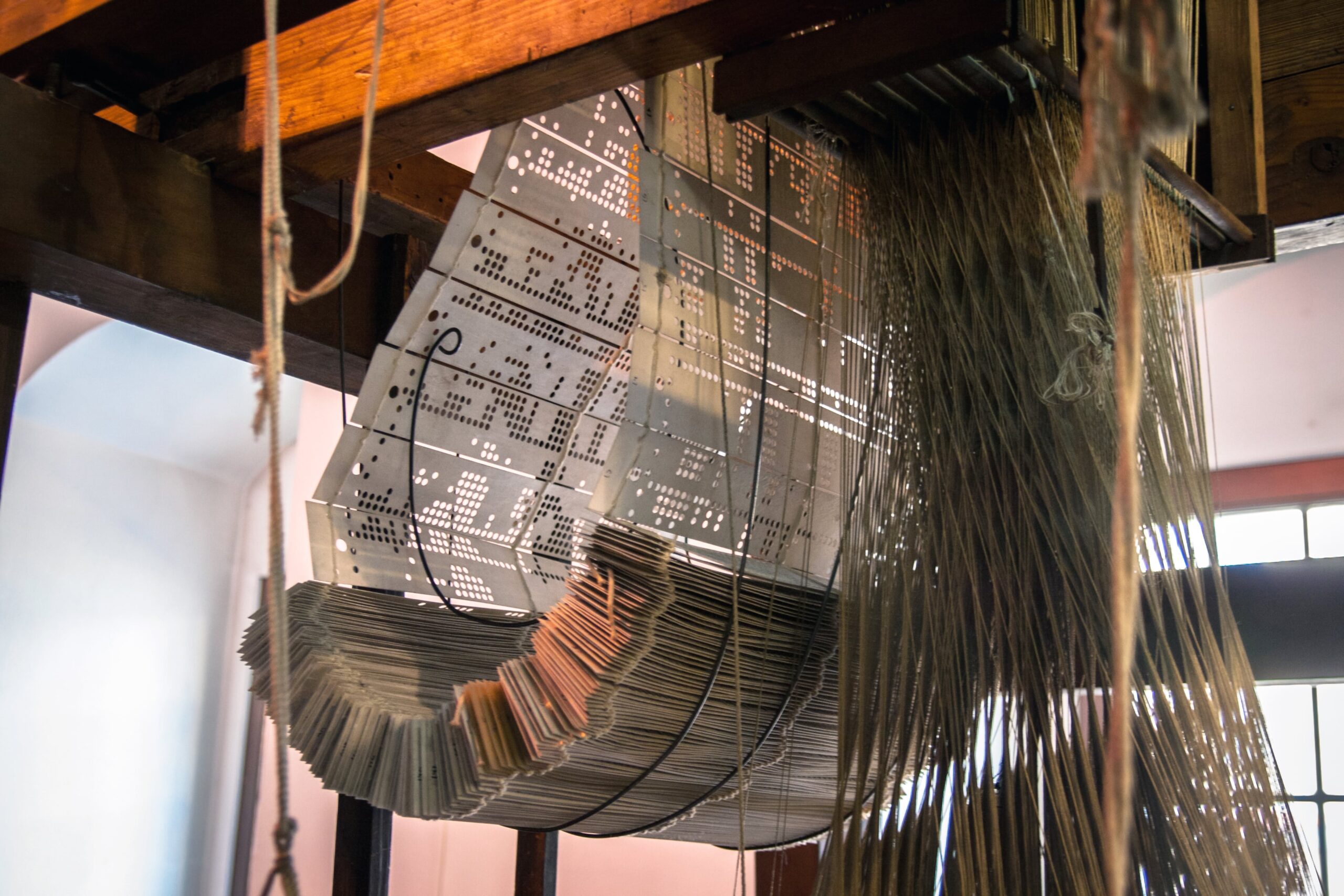
This guide to all things related to CNC machining will walk through how modern CNC machining processes work, including material options, design considerations, cost drivers, and more. Keep reading or click here to download the PDF.
Types of CNC Machining
Today, there are several technical processes included underneath the CNC machining umbrella.
3-Axis Machining: Milling and Turning
Once the operator enters the machining instructions, 3-axis CNC machines will carry out the input operations by using cutting tools to cut along three axes: X (left to right), Y (front to back), and Z (up-and-down). CNC milling and CNC turning are two common examples of 3-axis machining.
3-axis CNC milling involves fixing the block of material — also called the workpiece or “blank” — with a vice or machine bed. This holds the workpiece stationary as cutting tools and rotating drills, attached to a central spindle, make cuts to remove material and shape the final component. The 3-axis CNC milling machines are easy to program and operate and can be used to create most parts with simple geometric designs.
Since the cutting tools and drills are limited to three axes, some design features or areas of the blank may be difficult to complete or reach (e.g., unconventional shapes or deep, narrow cavities). However, multiple setups can help orient the workpiece for the machines to reach these features. While almost every 3-axis machining job has more than one setup per part, too many setups per part can quickly increase production costs. Processing geometrically complex parts can, for instance, require operators to manually reposition workpieces, which can impact processing speed, increase labor-related costs, and create more opportunities for human error.
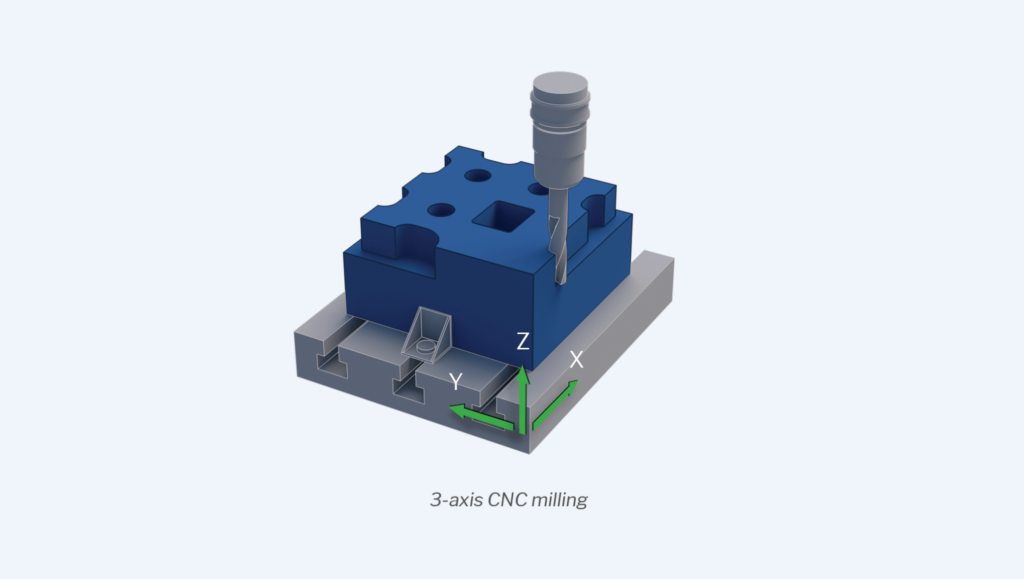
The CNC turning process operates a little differently. The blank is fixed to a rotating spindle, and a lathe then shapes the piece’s inner and outer perimeters or bores holes around the workpiece’s center axis. The most significant design restriction with CNC lathes is that they always produce rotationally symmetrical parts. The process is ideal, therefore, for the manufacturing of parts such as screws, bowls, or chair legs. For these types of rounded parts, lathes can produce pieces faster and at lower costs than CNC milling machines, especially when producing parts in high volumes.
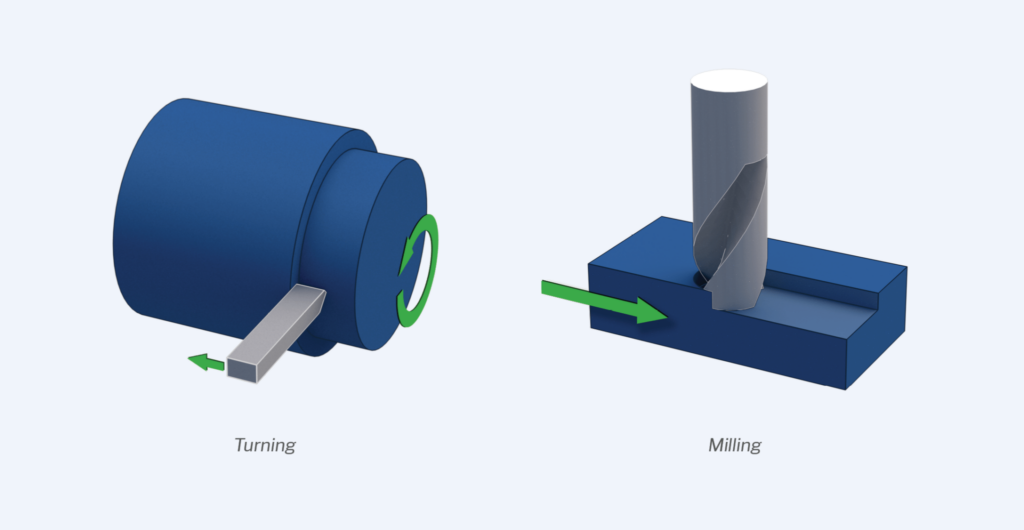
5-Axis or Multi-Axis Machining
Multi-axis machining processes allow the cutting implements greater freedom of movement while the workpiece is milled or turned. 3-axis processes allow either the blank or the cutting tool to rotate, but not both. In contrast, 5-axis processes allow both the workpiece and the tool to rotate and move along the X, Y, and Z axes simultaneously. 5-axis CNC machining saves time and is ideal for creating complex and precise parts like those found in the medical, oil and gas, and aerospace industries.
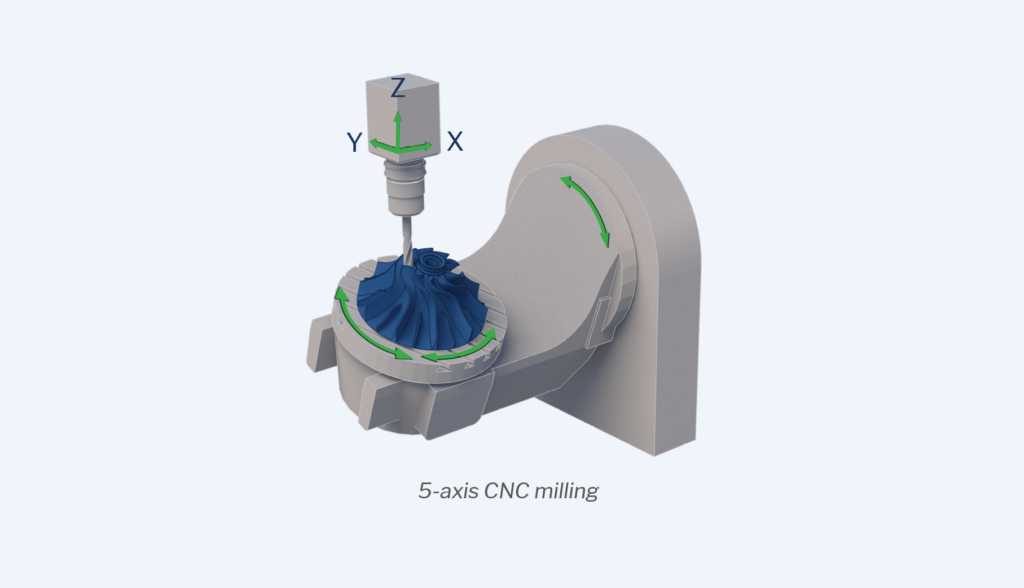
The multi-axis CNC machining family includes three processes:
- Indexed 5-axis CNC milling: During indexed 5-axis milling operations, not only can the cutting tools move along the three linear axes, but between operations, the machining table and tool head can swivel in two directions. The most noteworthy benefit of 5-axis milling is that the blanks no longer need to be manually adjusted between cutting operations, which makes it possible to create components with complex geometries more accurately and with greater efficiency. Indexed 5-axis machining is great for fabricating components like housings, jigs, and fixtures. The process falls somewhere between 3-axis CNC milling and continuous 5-axis CNC machining (see below) in terms of speed, precision, and the ability to handle complex geometries.
- Continuous 5-axis CNC milling: Continuous 5-axis CNC milling machines can move and rotate both the cutting tool and the workpiece simultaneously during each operation, allowing for the manufacture of parts with extremely intricate geometries and smooth surfaces. While this is the most expensive form of CNC machining on a per-part basis, the cost of continuous 5-axis CNC milling is typically offset by improved surface finish, speed, and dimensional stability.
- Mill-turning machining with live tooling: Mill-turning combines elements of CNC lathe machines with milling tools. The workpiece is affixed to a rotating spindle while cutting tools remove material from the blank. By combining the elements of CNC lathe machines with milling tools, mill-turning CNC offers high levels of accuracy and geometric versatility, making it great for creating parts with loose rotational symmetries, such as camshafts or centrifugal compressors.
Choosing the Right Type of CNC Machining
When selecting a manufacturing process for your part, you’ll need to evaluate if it’s a better fit for 3-axis or 5-axis machining. Generally, parts with simpler designs can be made quickly and affordably using 3-axis machines, while 5-axis machines are better for creating geometrically complex pieces with increased speed and accuracy.
If you’re on a budget or only need to cut a flat surface, 3-axis machines are the way to go. In addition to being more affordable, 3-axis machines are simpler to program, so you won’t have to incur the cost of working with expensive 5-axis programmers and operators. Plus, prep time is shorter with 3-axis machining.
If you need to produce a deeper part or one with complex geometry, you’ll want to use 5-axis machining. Using 5-axis machines lets you machine the workpiece from all sides with no manual rotation required. With 5-axis machining, you’ll have higher yields, greater accuracy, and increased freedom of movement, as well as the ability to manufacture larger parts faster.
Common CNC Materials
One of the advantages of CNC machining is that the process is compatible with many different materials, plastics and metals being the two most common material families. Here are some of the plastics and metals you’re likely to encounter.
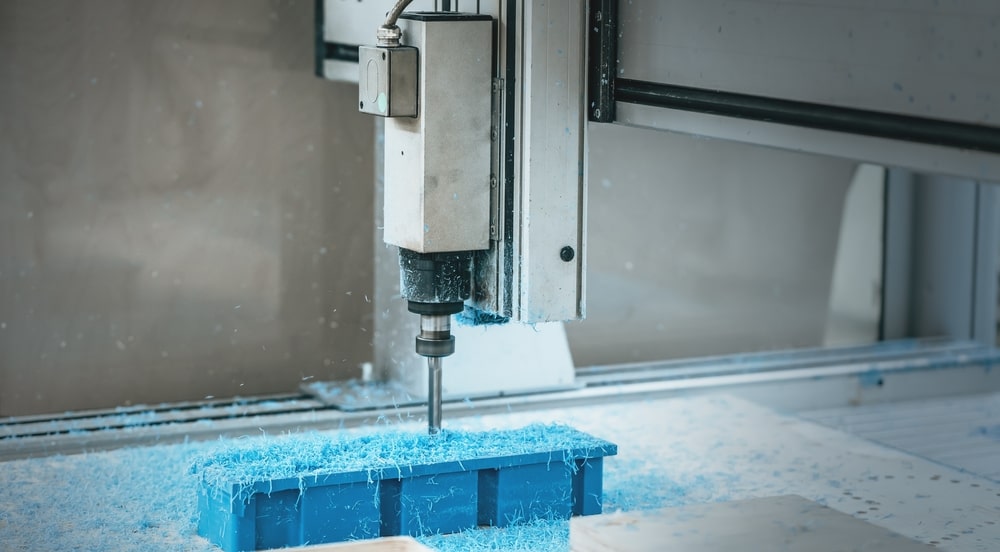
High-Performance Polymers
- Acrylonitrile butadiene styrene (ABS): ABS is an impact-resistant engineering thermoplastic with excellent mechanical properties. Strong and rigid while retaining a degree of flexibility, ABS is noteworthy for being mechanically strong, providing good insulation, and being resistant to abrasion and strain.
- High-density polyethylene (HDPE): One of the most versatile thermoplastic polymers around, HDPE is a flexible and easy-to-process material that is highly resistant to stress cracking, chemicals, and corrosives — even at low temperatures. It also offers excellent impact strength. HDPE is commonly used to make plastic bottles, plugs, seals, insulators, piping, and many other goods.
- Low-density polyethylene (LDPE): Primarily used to create orthotics and prosthetics, this tough, flexible plastic is easy to weld and heat seal. While providing good chemical resistance, LDPE is not ideal for applications that require stiffness, high structural strength, or high-temperature resistance.
- Polyamide (PA) or Nylon: Nylons are a family of low-friction plastics that are well-suited for replacing metal components, like bearings or bushings, due to its unique combination of elasticity, rigidity, and stiffness. Nylons can be reinforced with a range of additives to create specific material and chemical properties or combined with other plastics for increased performance and material characteristics.
- Polyamide (PA) or Nylon 30% GF: Glass fibers, one of the most common additives for engineering plastics, dramatically increase the strength and rigidity of nylon parts. Unfortunately, these fibers also increase the brittleness of the material and are therefore recommended only for applications that do not involve high-impact stress or deflection.
- Polycarbonate (PC): This plastic is widely used for a number of reasons — it’s tough while still being lightweight, it’s a good electrical insulator, and it’s naturally heat resistant. PC is inherently transparent and translucent and takes on pigment well. In addition, PC is resistant to diluted acids, oils, and greases, but is susceptible to hydrocarbon wear and UV rays.
- Polyetheretherketone (PEEK): A high-performance polymer with a unique combination of mechanical properties, PEEK is chemical-, wear-, creep-, fatigue-, liquid-, and temperature-resistant. In addition to being recyclable and biocompatible, this material is also lightweight, strong, and insoluble in all common solvents. Similar to nylon, PEEK can replace metal in CNC machining. Common applications for this material include piston units, critical airplane engine parts, and dental syringes. Notably, it is one of the more expensive CNC machining plastics on the market.
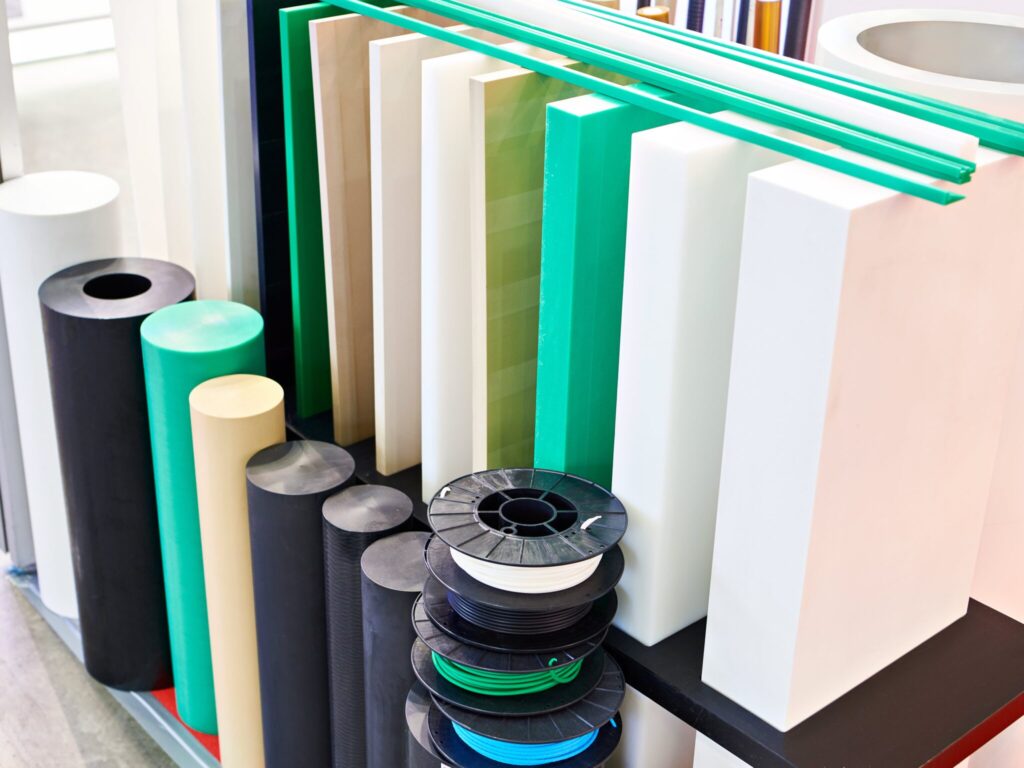
- Polyetherimide (PEI): Commonly known as ULTEM®, PEI is a high-performance plastic and manufacturing staple. Not only is PEI strong and easy to use, but it’s also resistant to chemicals and flame, and can withstand extremely high temperatures without losing its stable electrical properties. For this reason, PEI is typically used to create products like circuit boards, eyeglasses, food preparation and sterilization equipment, and aircraft parts. It’s one of the few commercially available amorphous thermoplastic polymers that keeps its mechanical integrity at high temperatures.
- Polymethyl methacrylate (PMMA): PMMA is a rigid thermoplastic polymer often called acrylic or acrylic glass. Extremely shatter-resistant, this tough and lightweight material is translucent and resistant to weathering and UV light, making it ideal for low-stress applications like greenhouses, the covers of car lights and turning signals, or solar panels. However, it’s important to note that PMMA is susceptible to high heat, impact, wear, and abrasion, and it’s prone to cracking under heavy loads.
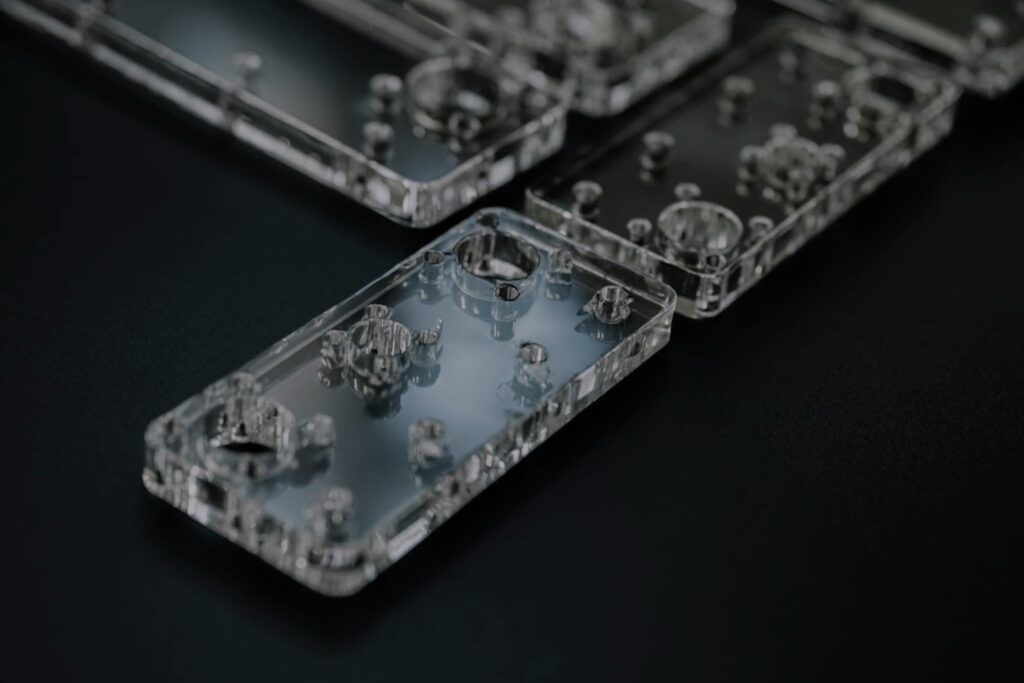
- Polyoxymethylene (POM): Commonly known as Acetal or by its branded name, Delrin®, this semi-crystalline engineering-grade thermoplastic offers excellent dimensional stability, stiffness, and low friction. Due to these properties, acetal is often used to create highly precise parts used in applications where high strength, durability, and wide operating temperature range (-40°C to 120°C) are required.
- Polypropylene (PP): This cost-effective plastic is lightweight, highly resistant to chemicals in corrosive environments, and resistant to most organic solvents, degreasing agents, and electrolytes. Compared to LDPE and HDPE, PP has a lower impact strength but offers better tensile strength.
- Polytetrafluoroethylene (PTFE): PTFE, commonly known as Teflon®, is a versatile polymer favored for its mechanical properties. Resistant to chemicals, temperature, light, UV rays, water, weathering, fatigue, and adhesion, PTFE is commonly found in the coatings of nonstick pans but is also used in semiconductors, gaskets, and even some medical applications. Although PTFE is one of the most corrosion-resistant plastics, it’s sensitive to creep and abrasion.
- Polyvinyl Chloride (PVC): PVC is one of the world’s oldest plastics. Stark white and rigid, PVC is an affordable rubber alternative that has been in high demand for decades. Boasting strong resistance to light, chemicals, and corrosion, PVC is a popular material in the healthcare, IT, transportation, textiles, and construction sectors.
- Ultra-high molecular weight polyethylene (UHMW): A tough, versatile, and highly machinable plastic, UHMW can be used to create parts with high abrasion and wear resistance, durability, and low friction coefficients. UHMW isn’t typically suited for high load-bearing applications or conditions exceeding 80 to 100°C.
Metals
Steels and stainless steels, aluminums, and soft metals like brass, bronze, titanium, and copper are among the most popular machinable metals. While steels and aluminums are the most commonly machined, each family of metals offers a different set of physical properties and price points.
- Aluminums: Aluminums are soft, low-density, non-magnetic metals that are much easier to machine than steel. Due to their low tensile strength, these metals are often alloyed with other materials in order to accentuate desired physical properties. Aluminums are generally around 1/3 the weight of steel, meaning that they offer a better strength-to-weight ratio. This can be useful in lightweighting parts. They are also much better thermal and electrical conductors than steels.
- Steels: Steels are iron-based metals that have been alloyed with carbon and other metals, typically including manganese, phosphorus, or sulfur. Steels tend to be stronger and more durable than aluminums. However, they are also heavier and harder to machine without the use of carbide or other specialty tools, which can increase production costs.
- Stainless steels: The primary difference between steels and stainless steels is that the latter is alloyed with chromium rather than carbon. While standard steels are susceptible to rust and corrosion when exposed to moisture and oxygen, the chromium content in stainless steels creates a passive chromium-oxide layer on the surface of the metal, preventing the iron within from oxidizing further. This also inhibits steels’ ability to conduct electricity. Stainless steels can be alloyed with other metals (including molybdenum, nickel, or titanium) to increase a part’s resistance to corrosion and heat.
- Soft metals: Soft metals like brass, bronze, titanium, and copper offer a wide range of physical characteristics that are attractive for specific applications. However, soft metals — especially those with high copper content — may actually be more difficult to machine due to copper’s high ductility. Machining soft metals can contribute to increased heat build-up at the cutting site, greater tool wear, and galling (a gummy build-up on the tool’s cutting edge). Titanium, specifically, is also less rigid than other metals and therefore requires a tight grip on the workpiece to ensure precision and accuracy. All of these factors can contribute to higher machining costs for soft metals.
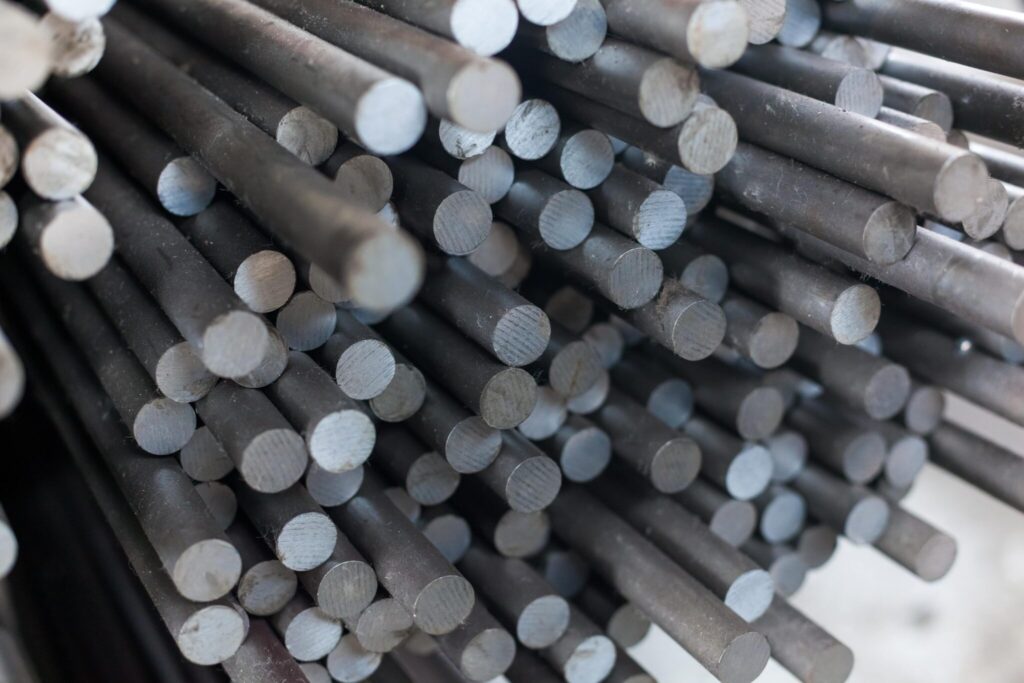
CNC Surface Finishes and Post-Processing
After a part or component has been machined, it may need to undergo one or more finishing processes. These processes can be used to remove aesthetic flaws, improve a product’s appearance, provide additional strength and resistance, adjust electrical conductivity, and more. Common finishing processes for machined parts are anodizing, powder coating, and bead blasting. However, it is also common to leave parts as-machined or as-milled when other finishes are not necessary.
Common CNC Finishing Options
- Anodizing (aluminum): Anodizing is an electrochemical process in which aluminum or titanium alloys are submerged in an electrolytic bath in order to thicken a machined part’s natural oxide layer to make it denser, electrically non-conductive, and more durable. Anodized finishes also promise good dimensional control, which makes them best used in high-performance engineering applications, particularly for internal cavities and small parts. Anodizing offers one of the most aesthetically pleasing finishes for CNC machined parts, but it comes at a higher price.
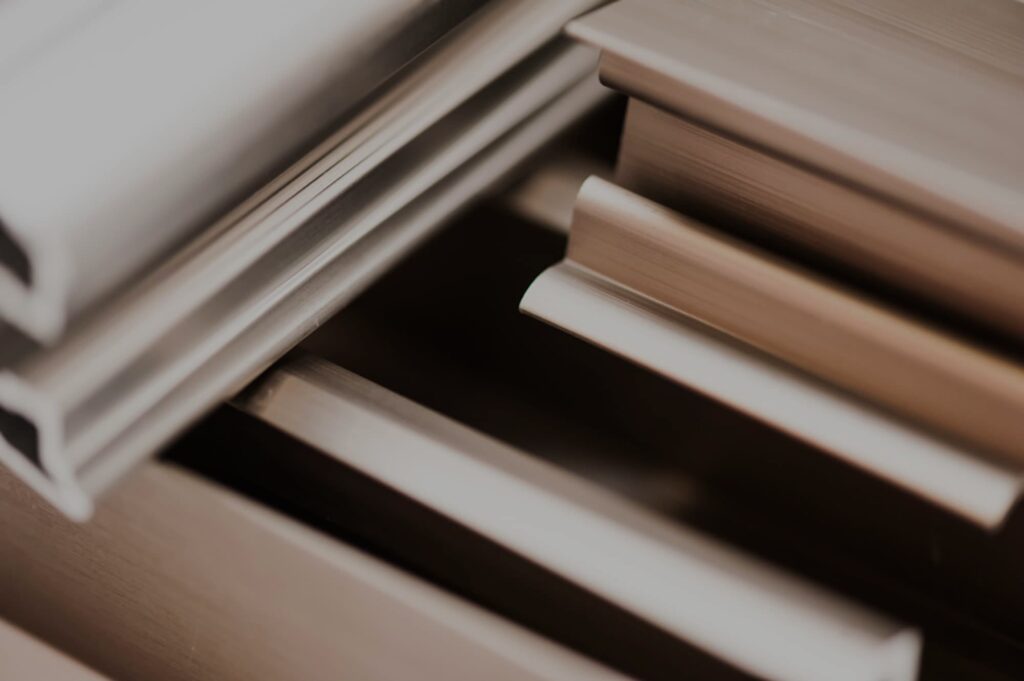
- Bead blasting (metal or plastic): Bead blasting uses a pressurized air system to fire millions of glass beads at the part, which effectively removes tool marks and imperfections, and can be used to create a consistent grainy, matte, or satin surface finish. Bead blasting adds no chemical or mechanical properties and, unlike powder coating which adds material to a part, it’s a reductive finish, meaning that the process removes material from the part. This is an important consideration if your part has strict tolerances. Bead blasting is one of the most affordable surface finishes but must be executed manually. Bead size and grade will also affect the final finish.
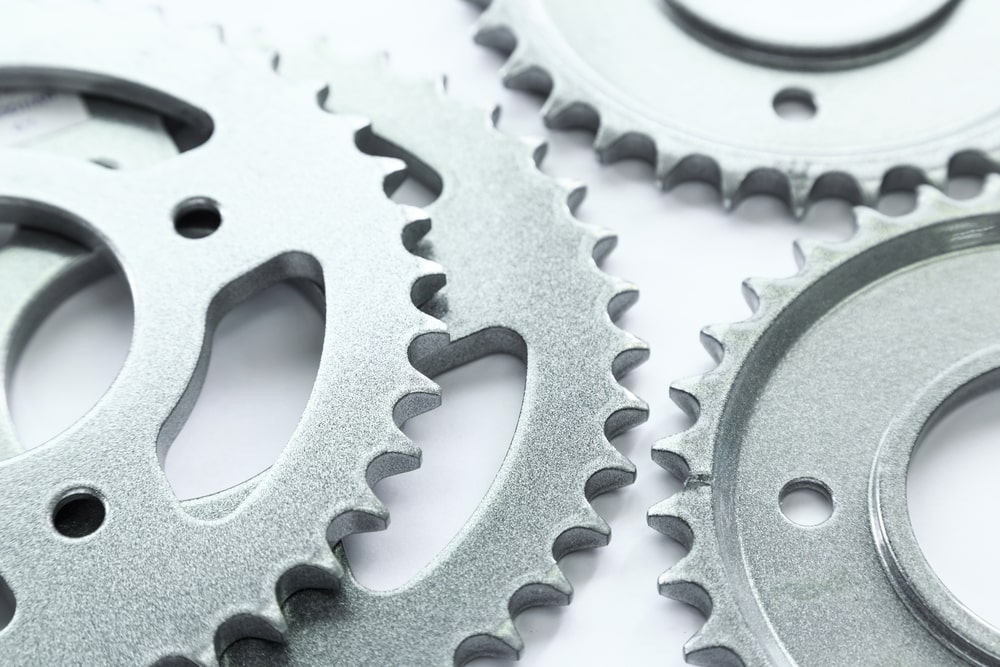
- Black oxide (steel, stainless steel, copper): This electrochemical or chemical treatment process creates black iron oxide on the surface of ferrous metals. Also called blackening, oxidizing, or black passivating, this process does not simply deposit a black oxide layer on the surface of the metal. Rather, the chemical reaction between the iron in the metal and the oxidizing salt solution creates an iron oxide called magnetite, which has a matte black appearance. This process improves the part’s dimensional stability and aesthetic appearance while reducing the surface’s light reflection, which is important for parts used in applications involving radiation. Black oxidizing steel can also help sharpen tools like screwdriver tips and drill bits.
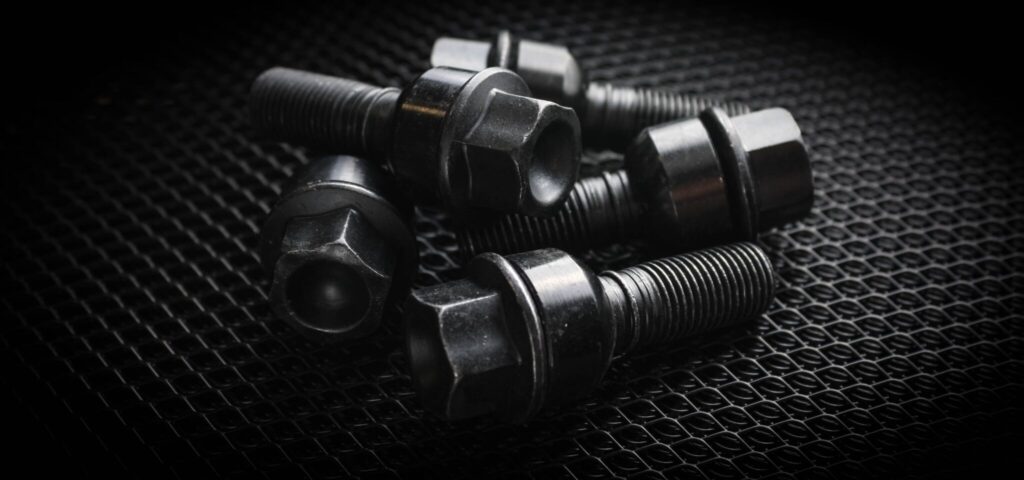
- Powder coating (all metals): Powder coating is similar to spray painting. The machined part receives a primer coat to protect it against corrosion. Then, the part is “painted” with a dry powder coating from an electrostatic spray gun and cured in an oven heated to at least 200°C. Multiple layers can be applied to increase the thickness of the finish, which creates a thin protective layer on the part that is strong, wear-resistant, and aesthetically pleasing. This process can be combined with bead blasting to increase the part’s corrosion resistance and create greater uniformity in texture and appearance. Unlike anodizing, a powder-coated finish is compatible with all metals, is less brittle, and offers greater impact resistance. However, powder coating generally yields less dimensional control than an anodic finish and is not recommended for use in small components or internal surfaces. Powder coating’s higher price point can also make larger production runs expensive.
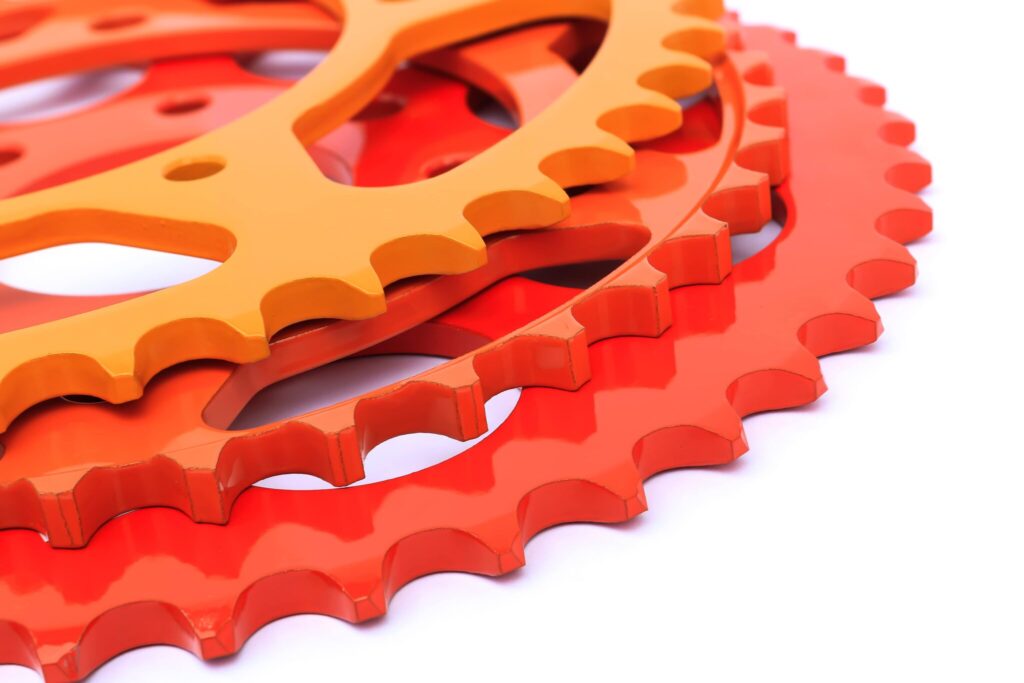
- As-machined (metal or plastic): Not applying finishing processes to machined parts is referred to as an “as-machined” or “as-milled” finish. The part will have small but visible tool marks and blemishes. As-machined parts have the tightest dimensional tolerances and are extremely affordable to produce because post-processing isn’t necessary. This is ideal for applications in which dimensional integrity matters more than aesthetics. However, it’s important to note that without additional finishing or protective coating, the surface hardness of as-machined makes them susceptible to nicking, scuffing, and scratching.
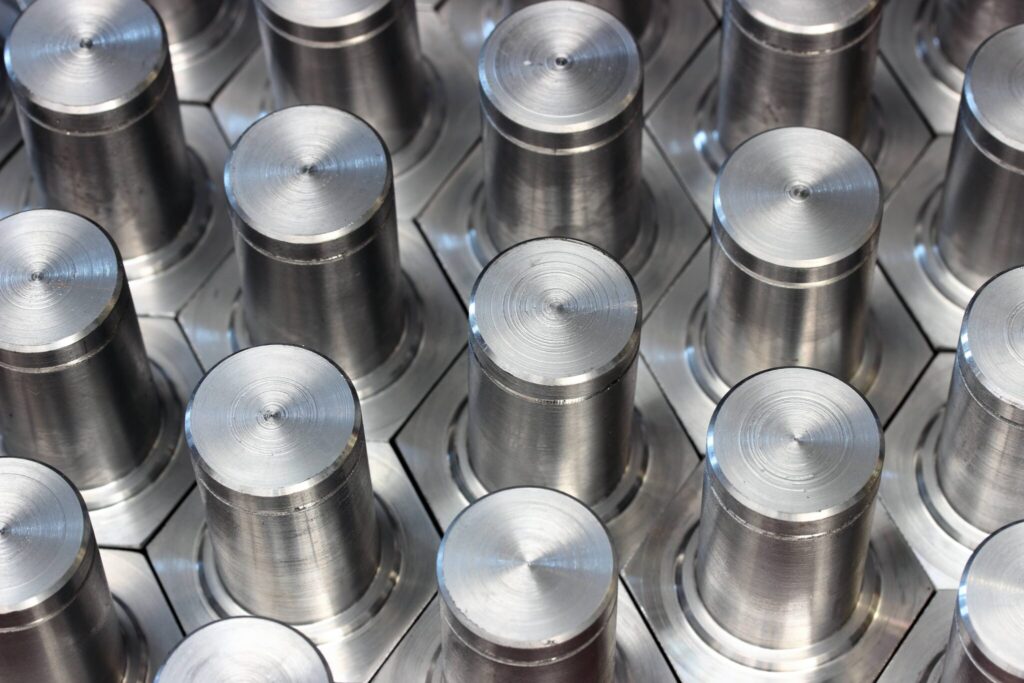
Choosing the Right Materials and Finish
Selecting materials and post-processing options for your part relies heavily on how and where your part will be used. Key end-use considerations for selecting your part material and surface finish include:
- Environmental factors: The environmental conditions of a given part’s end-use application play a significant role in determining which materials and treatments are ideal. Factors like heat, cold, flame, UV radiation, exposure to chemicals or autoclaving, and more must be taken into account in order to ensure the viability of the part.
- Electrical factors: Whether a part requires conductive or insulating properties is another critical consideration. Knowing your part should conduct electricity, you may select a conductive material like copper, whereas a material like Nylon 66 would be appropriate when searching for a good insulator.
- Mechanical factors: Parts intended to endure heavy loads, wear, or other external forces need to be made from materials that can withstand those forces. Identifying the most desirable or necessary properties for a part — such as flexibility, impact resistance, tensile or compressive strength — can help teams pinpoint which materials make the best fit.
- Cosmetic considerations: While aesthetics can be of secondary priority for some parts, this is not the case for many consumer products. Some parts or applications may require materials with particular cosmetic options when it comes to color, transparency, or surface finish.
- Tolerances: Some degree of variation between parts is expected in manufacturing, and dimensional tolerances refer to the range of acceptable variation that viable parts must fall within. Tighter tolerances are more labor-intensive and costly to achieve repeatedly but may be necessary based on how a component is used.
Designing for CNC Machining
Designing a high-quality part that will perform as expected is only half the challenge. You must also be able to machine the part in an efficient and cost-effective way. Design for manufacturability (DFM) is the process framework that helps integrate how a part will be made with the part design. DFM is critical to manufacturing and affects everything from production timelines and costs to operational efficiency and the quality of the part itself. The most common design considerations for CNC machining include:
Deep Pockets:
Features like deep or narrow pockets and slots require longer tools to machine properly. However, longer cutting tools are more prone to breaking and are often less precise due to machining vibrations, or chatter. Deep features also typically require several cuts to machine properly, which increases both machining time and production costs — especially since smaller tools will likely be necessary to complete the finishing passes. If slots or deep pockets must be machined, aim to either reduce the depth of the feature as much as possible or increase the area of the pocket’s cross-section. The depth of a pocket or slot should be no more than 3x the diameter of the smallest tool needed.
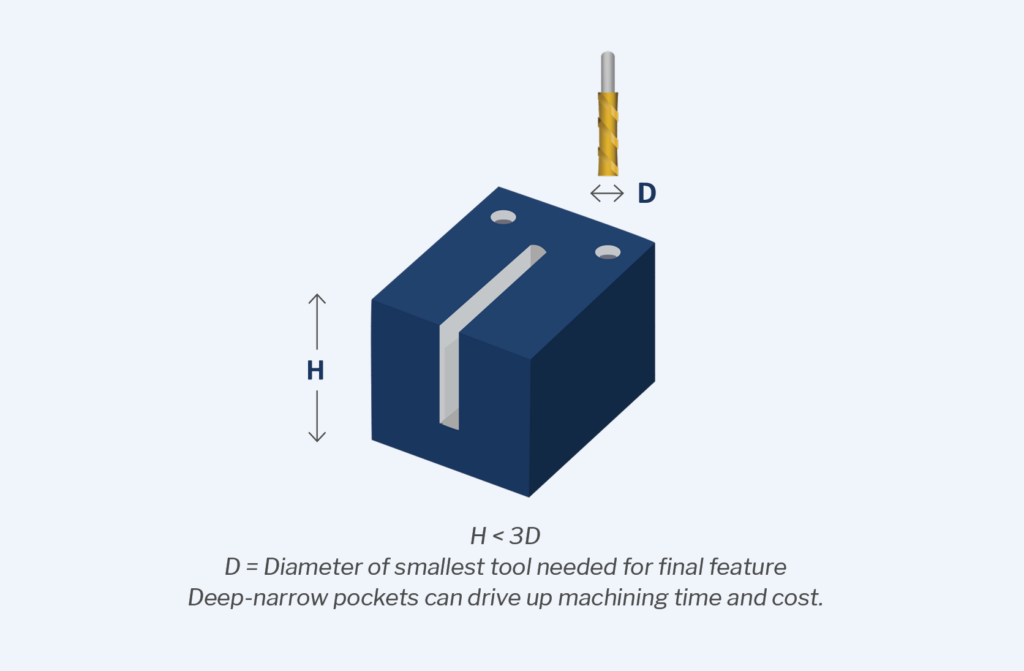
Narrow Regions:
Narrow features limit the size of viable cutting tools. This can present a challenge for manufacturers because of how susceptible long, small-diameter tools are to chatter and breakage. Just as with deep features, narrow regions should be no less than 3x the diameter of the smallest cutting tool. Minimizing the depth of these features allows you to use shorter, larger-diameter cutting tools, which helps to reduce machine chatter.
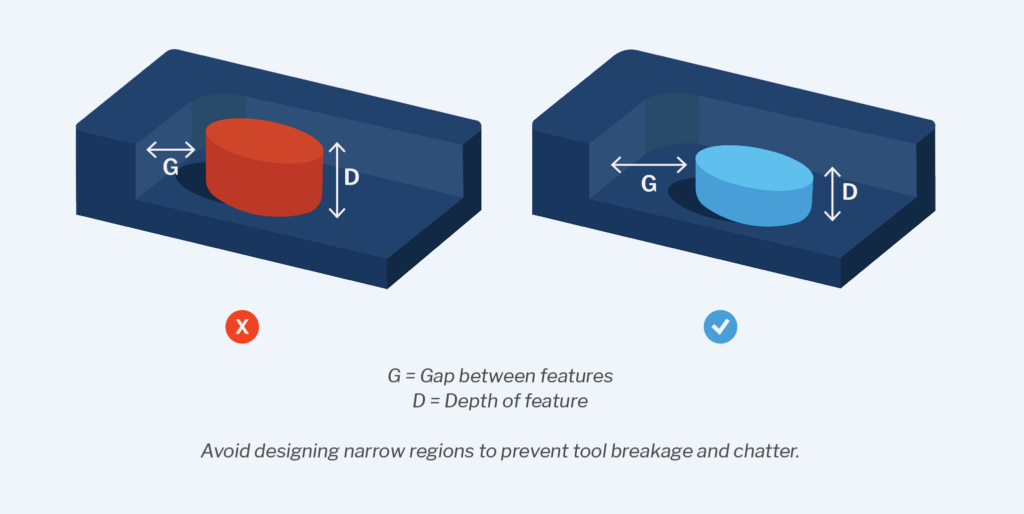
Sharp Internal Corners:
CNC end mills are circular, which makes it difficult to machine sharp internal corners because the bits will leave behind a corner radius. While there are methods of machining sharp internal corners, these processes tend to be costly. In general, sharp internal corners should be avoided for machined parts. Internal corner radii should also be larger than the radius of the intended cutting tool. By designing internal radii to be larger than the cutting tool, the machine can create a smooth transition between walls. In contrast, an internal radius that is the same size as the cutting tool will force the machine to make a sudden change in direction, resulting in a less smooth finish.
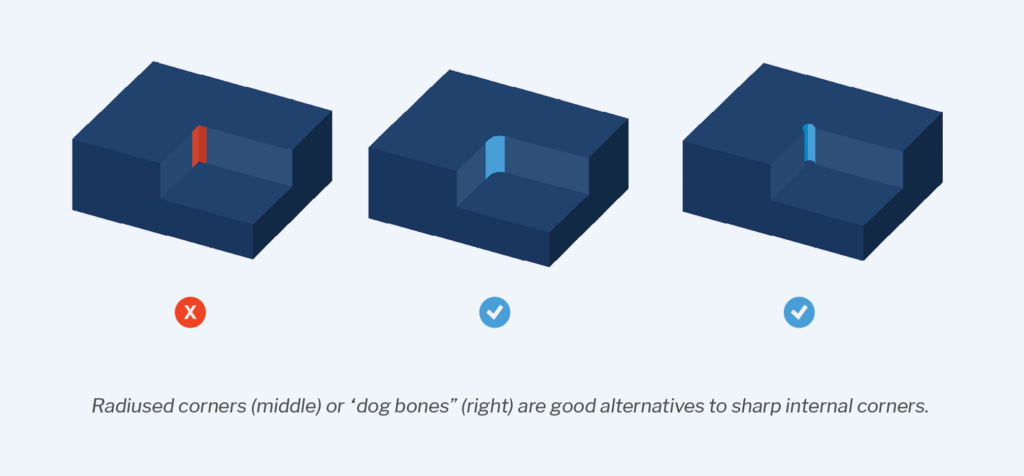
Inaccessible Features:
Features like counterbores — those that open inside another pocket or pockets with negative drafts — can pose a significant challenge for production teams. These design features take considerably more time to machine because of how difficult it can be to allow the cutting tool access. It’s critical to ensure that cutting tools can freely access all of a part’s features without being blocked by any other feature.
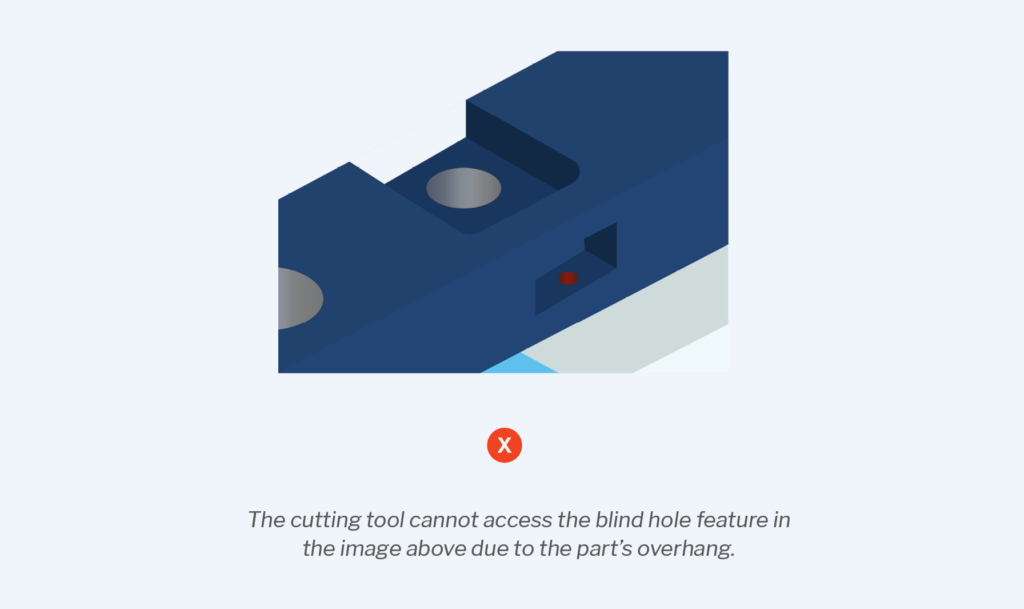
Outside Fillets:
Outside fillets, or rounded corners along the top edge of pockets, bosses, slots, and other features, require custom cutting tools supported by a precise machining setup — a pairing that can quickly become expensive. Beveling or chamfering the outside edges can help avoid these costs.
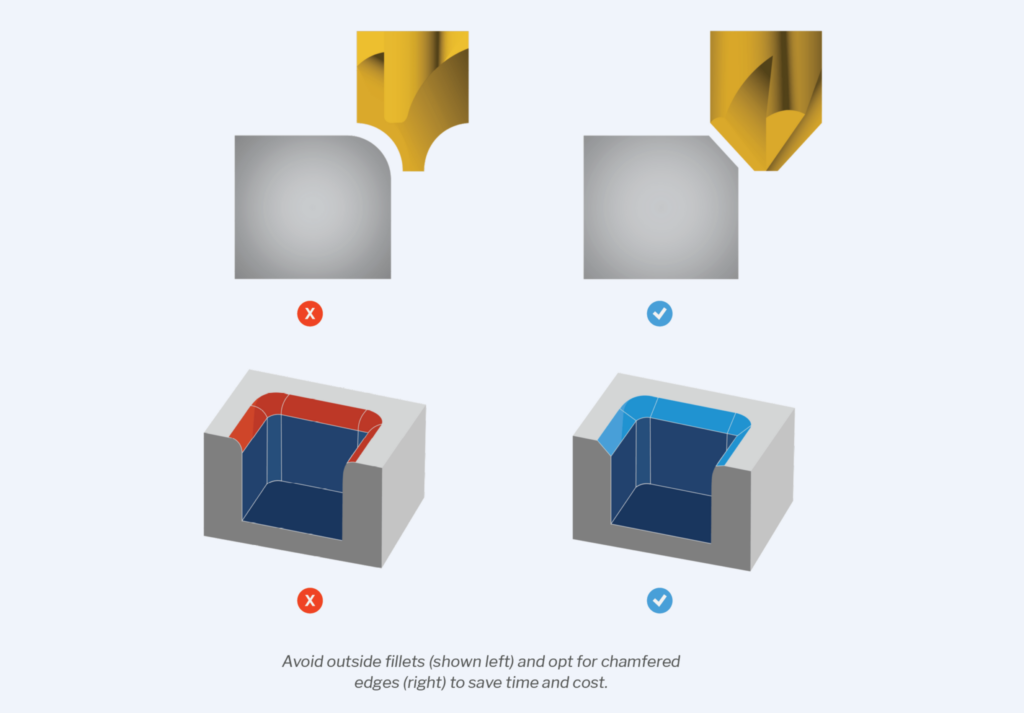
Wall Thickness:
Thin walls can create issues for metal and plastic parts alike and should be avoided wherever possible. Thin metal walls are more prone to chatter, for instance, which negatively impacts the accuracy of the part and its surface finish. Plastic parts with thin walls are also more likely to warp or soften. The minimum wall thickness for metal parts should be 0.03” (0.762mm) and 0.06” (1.524mm) for plastic parts.
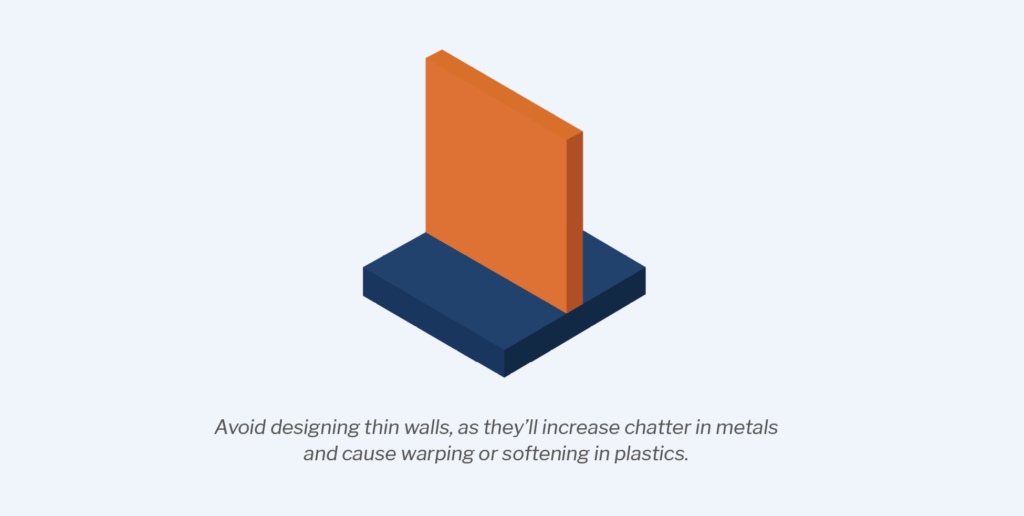
Flat-Bottomed Holes:
Holes with flat bottoms are not only difficult to machine, but they also tend to create difficulties for any subsequent operations. In general, product teams should avoid blind holes with flat bottoms in favor of standard twist drills, which create holes with cone-shaped bottoms.
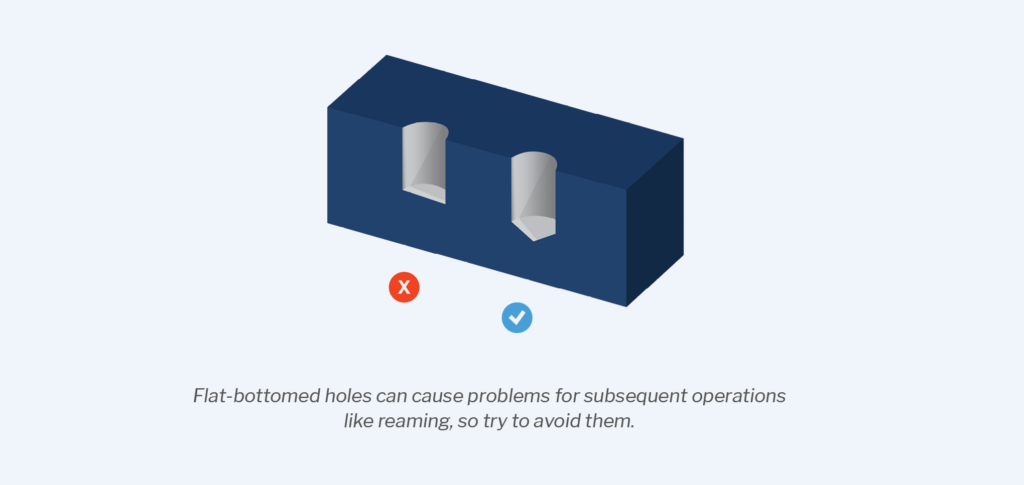
Threaded Parts:
When machining internal or external threads, there are a few different design considerations.
For internal threads:
- Include a countersink at the end of internal threads.
- Reduce the number of threads whenever possible. Most of a part’s stress actually falls on the first three threads, so increasing the number
- of threads produces diminishing returns.
- Use standard forms and sizes.
- Opt for coarse threads to keep costs low.
For external threads:
- Include a chamfer in all screw designs.
- Avoid terminating near the shoulder of areas with large diameters.
- Turned external threads should have a relief groove.
- Use shorter external threads unless the part specifically calls for high thread strength.
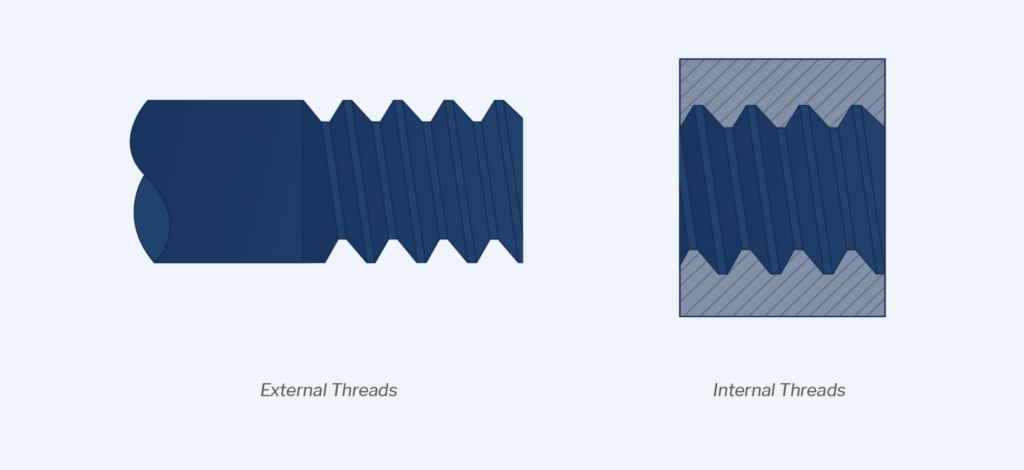
Drill Entrances and Exits:
If the surface of a workpiece is not perpendicular to the axis of a drill, the drill tip will wander when it comes into contact with the material. To minimize uneven exit burrs and streamline the burr-removal process, ensure that hole features have start and end faces that are perpendicular to the drill axis.
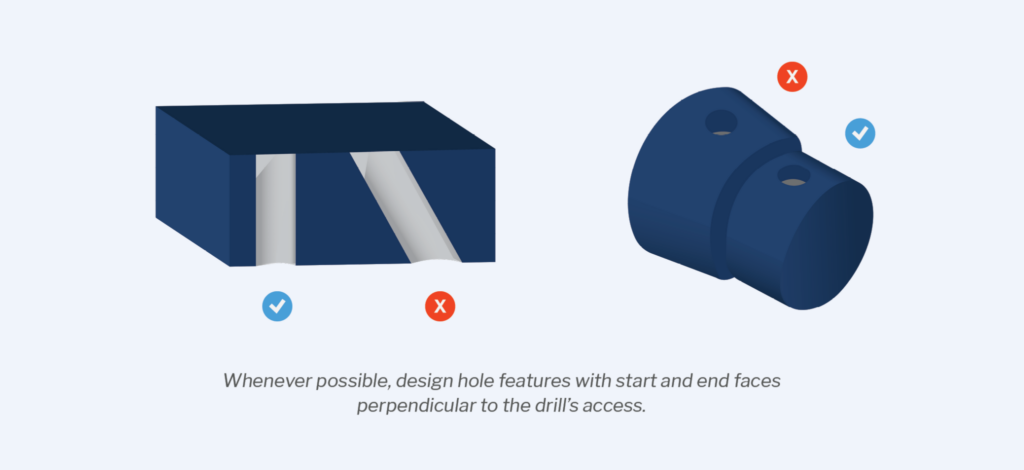
A Checklist for Reducing Costs
1. Keep parts simple
Parts with complex designs or manufacturing processes can have a number of downstream effects. Complexity can increase machining time and cost, introduce opportunities for error, and make it difficult for end-users to understand the exact use of components or parts. Separating which characteristics and design features are vital to the viability of the part from those that are desirable yet non-critical helps remove unnecessary complexity from a part’s design and machining operations.
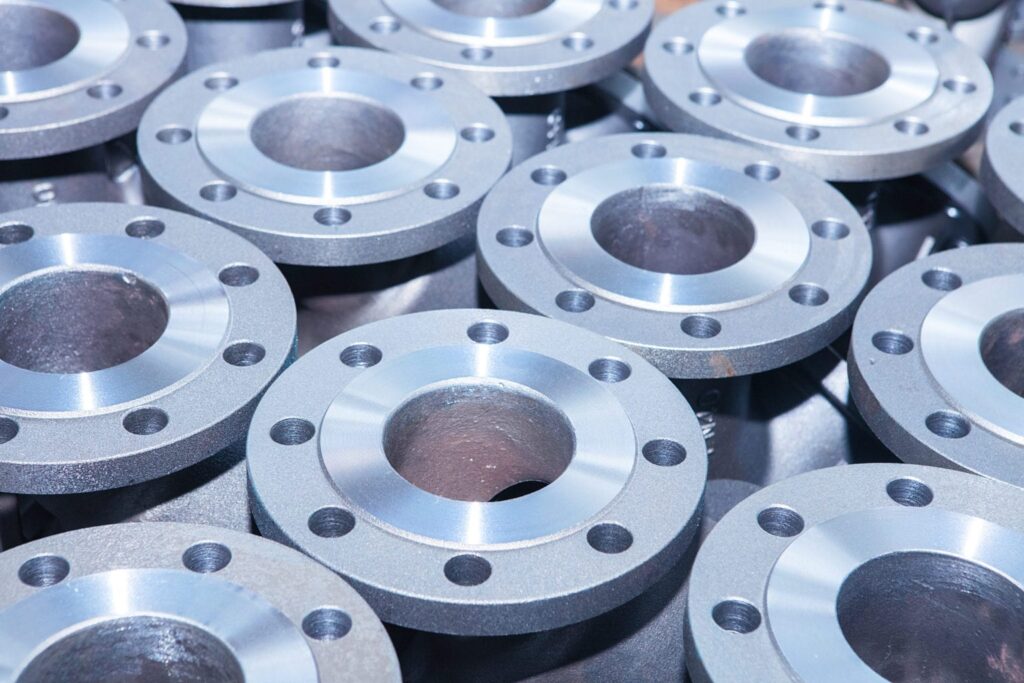
2. Design with tool geometry and setup orientation in mind
While most CNC machining jobs require more than one setup orientation, you’ll want to keep the number of orientations per part to a minimum. For example, if you need to flip a workpiece three times for the cutting tools to be able to access all of the features, this is going to increase costs because each setup requires re-mounting the part, zero-ing the machine to establish the correct axes, and running a new G-code program. 5-axis machines are going to be more capable in this aspect as they can reach more features without requiring multiple setups, though this is dependent on the geometry of a particular part.
Minimizing the number of cutting tools required is another way to reduce costs. As much as possible, try to keep radii consistent so that fewer tools are needed to machine internal corners. Likewise, avoid very small details where possible. Smaller cutting tools aren’t able to cut as deep into the workpiece and are also more prone to breaking. Typically, the smallest internal features that can be machined are 0.0394” (1mm), though holes can go as small as 0.0197” (0.5mm).
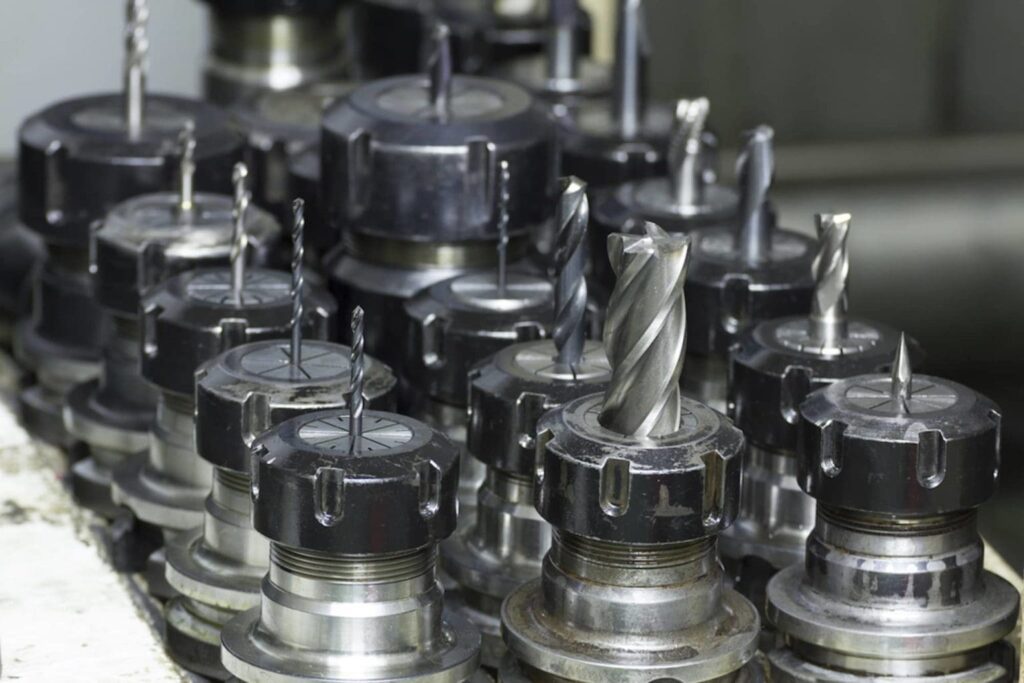
3. Balance your tolerances
Choose your critical dimensions thoughtfully. Not every dimension requires inspection or is critical to the viability of the part, so focus your attention on those that are most essential. Determining how precise specific features need to be will allow you to adjust tolerances accordingly, reduce costs, and streamline the manufacturing process. Make sure that tolerances are still within machineable limits, adhere to given standards, and account for tolerance stacking.
Avoid over-dimensioning your part, as well. Unclear part drawings defeat the purpose of geometric dimensioning and tolerancing, which is to clarify and streamline communication. At SyBridge, we recommend that you align drawing datums with the CNC coordinate system, as using consistent datum reference frames between machining and measurement systems is more likely to ensure parts are accurately located.
If possible, ensure all datums — points, surfaces, or axes used as references for measurement — reference the same setup. If a datum was machined in a previous setup, it becomes more difficult to hold tight tolerances after a setup change. Finally, be conscious about your datums. Choosing reference datums that are easy to measure from will ease manufacturing.
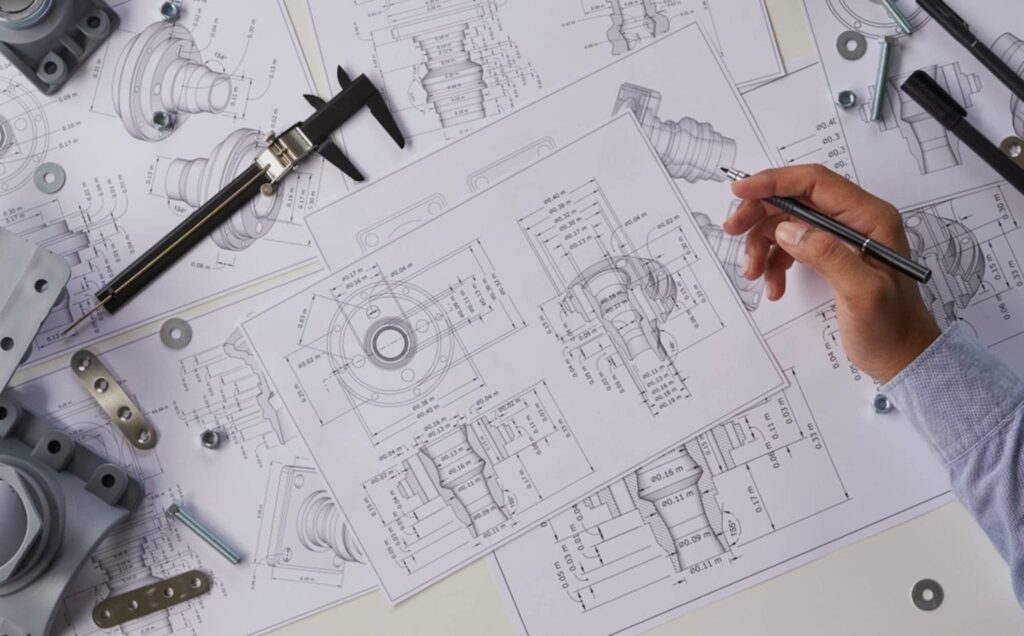
4. Tailor inspection levels
Similar to balancing your tolerances, strategically applying the right inspection levels can help reduce costs and optimize the production processes. Typical inspection options may include a visual inspection of the part, checking part dimensions using hand tools, or a first article inspection (FAI) where the first manufactured part is checked to ensure all requirements have been met. More in-depth inspections require more time and labor, which increases manufacturing costs.
Increasing the number of parts that you inspect will also increase the cost of production, so it’s important that you select the right sampling plan to ensure confidence across the entire order. At SyBridge, our default sampling rate is based on ANSI ASQ Z1.4 Level 2.
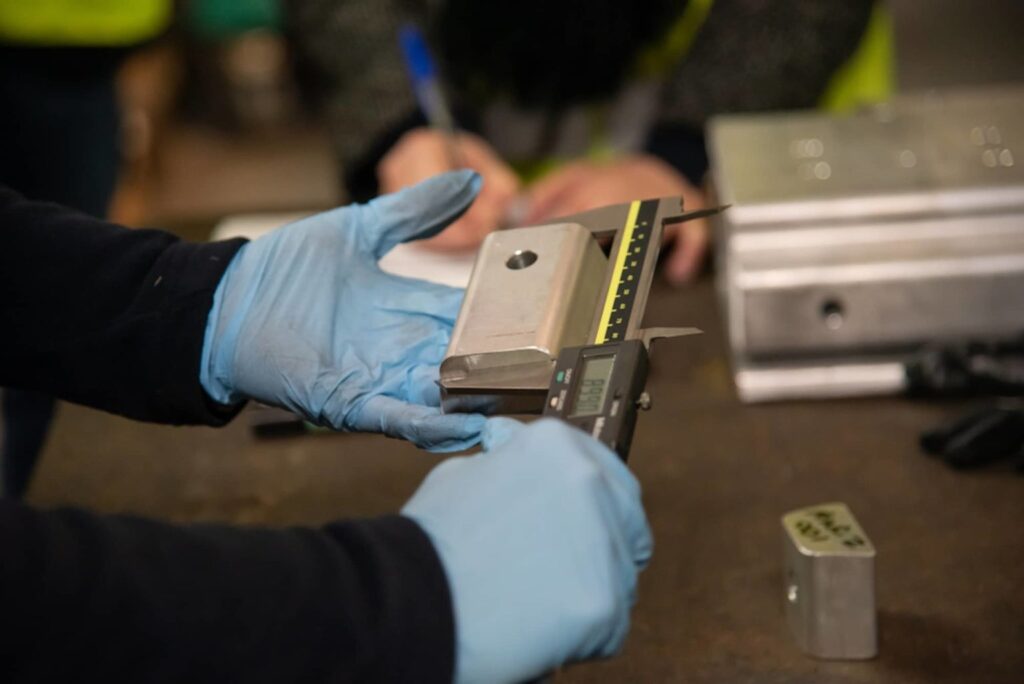
5. Design to common stock sizes
Designing parts to common stock sizes can help streamline the manufacturing process by minimizing the amount of material that needs to be removed from the workpiece. For example: if it’s acceptable for a part surface to retain its stock finish — that is, if the surface can be rougher than 125 μin Ra — and it does not need to be machined, you have an opportunity to reduce machining time without sacrificing part quality.
This is why knowing the common stock sizes for various materials is important. Metals, for instance, are typically available in a number of stock forms, including sheets, rods, bars, or tubes, which can be purchased according to dimensions like length, width, thickness, and diameter.
Keep in mind that metric sizes are more commonly available in Asia, while imperial sizes are more commonly available in the U.S.
6. Minimize deformation risk
Removing a large amount of material from a workpiece can cause the material to deform. This requires additional processing during machining to prevent deformation, leading to higher costs. Plastics have a higher risk of deformation than metals.
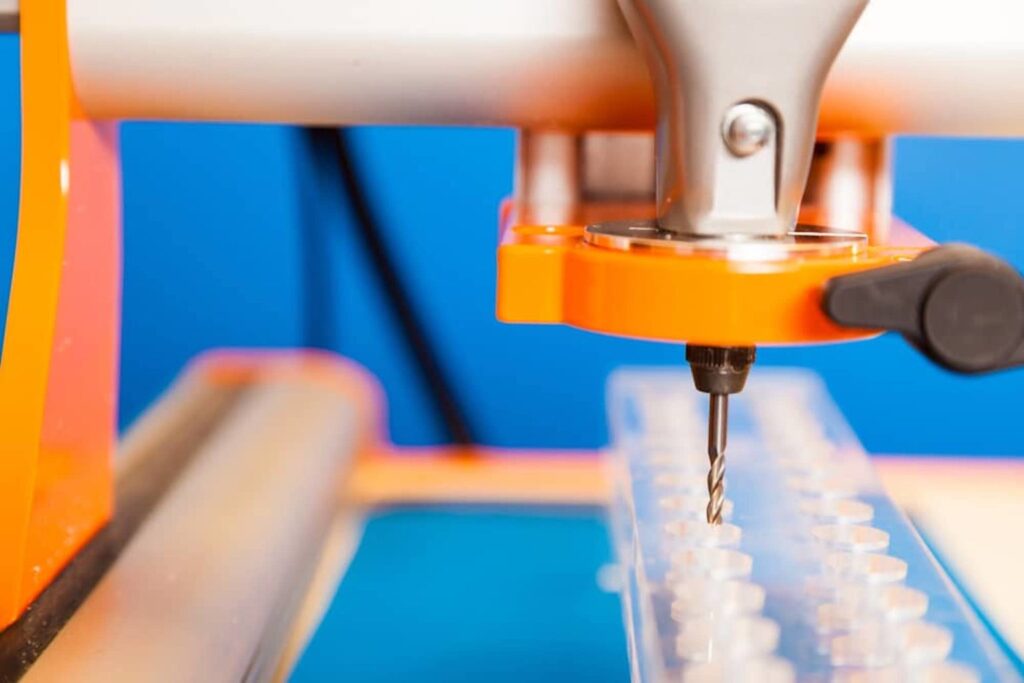
7. Choose your material carefully
The material you pick has a significant impact on the overall cost of your part. For example, metals are generally more expensive than plastics. Avoid over-engineering your parts and consider whether a specific grade or material certificate is required for the part. If a generic material can fulfill the part’s requirements or multiple materials can be accepted, select the less expensive material to keep costs low.
The machinability of the material should also be taken into consideration. Steels, for example, are typically more difficult to machine, which leads to higher costs because steel parts take longer to machine and increase wear-and-tear on cutting tools.
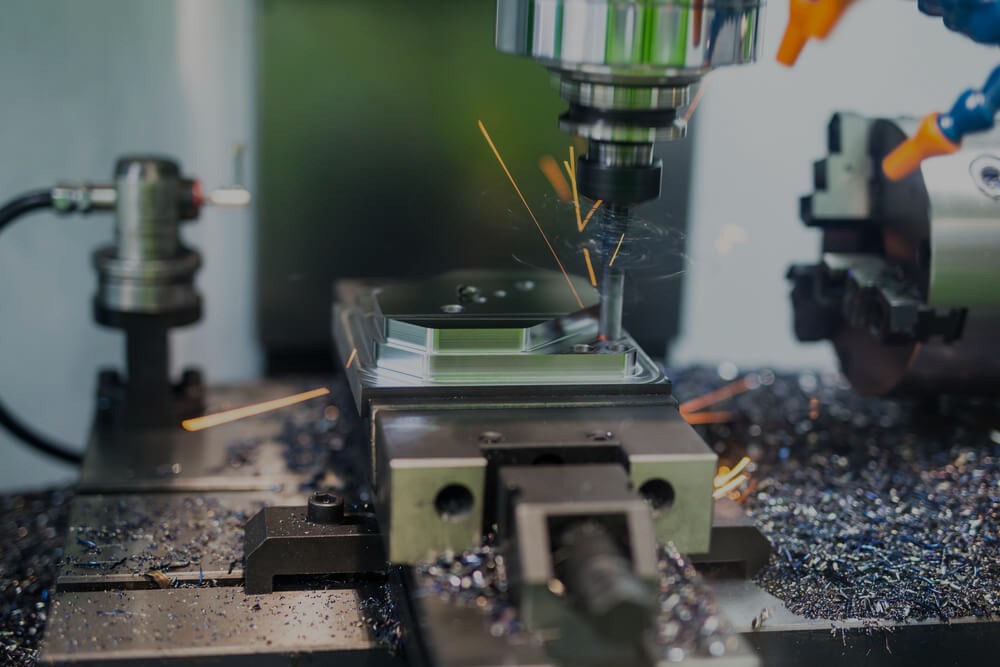
Common Applications for CNC Machining
Many industries rely on CNC machining processes to produce reliable, accurate parts that meet precise specifications and regulatory requirements, including the aerospace, automotive, medical device, electronics, and commercial parts manufacturing sectors.
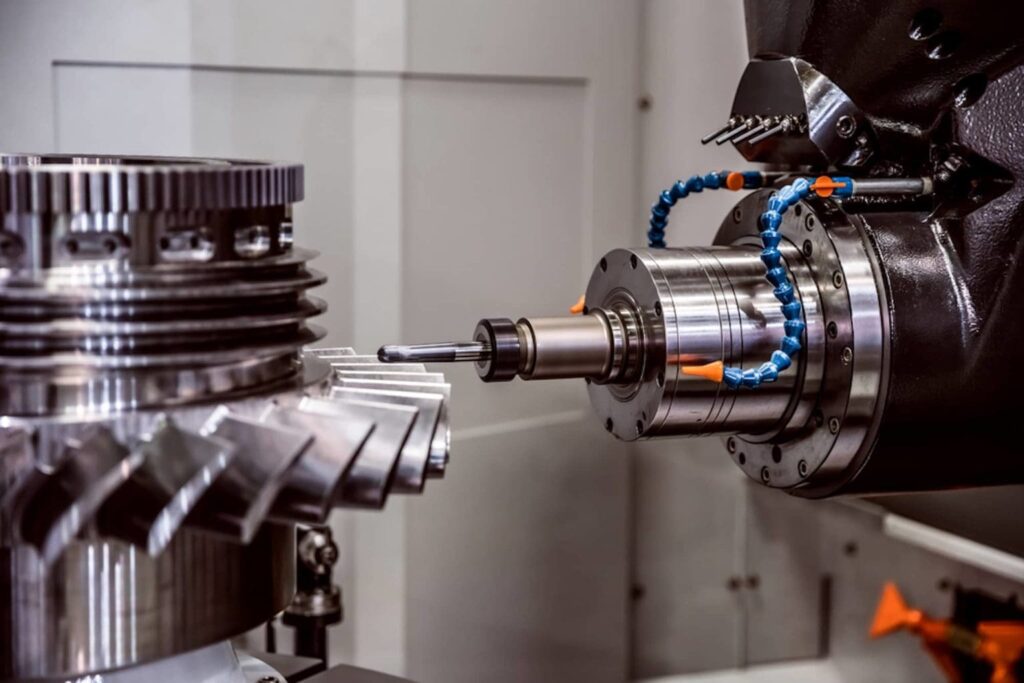
Aerospace parts are held to high quality, testing, and regulatory standards in order to ensure proper function, fit, and safety. CNC machining is an excellent fit for creating plastic and metal parts with extremely tight tolerances that satisfy the aerospace manufacturer’s need for an incredible degree of precision and a range of highly specialized parts. The same is true for automotive components.
CNC machining can also be used to create a variety of medical devices, from implants to surgical implements to components for medical electronics.
Care and precision are critical when producing these sorts of devices and tools, as they are held to additional safety standards and requirements. Semiconductors and electronics components also have incredibly stringent precision requirements and tolerance standards, given their size and complexity. As a process, CNC machining has few material limitations, allowing it to be used with conductive materials like silicon. Many commercial parts — from aluminum castings and extrusions to steel and plastic parts — can also be machined.
Another common application for CNC machining is tooling, or the process of creating the various components, tools, machinery, and master patterns that will be used in production. Tooling is an integral part of every manufacturing and molding process and encompasses items like molds, jigs, and fixtures.
Starting Your CNC Machining Project With SyBridge
At SyBridge, we make it simple and straightforward to get quality parts through our CNC machining service. To get started, visit os.fastradius.com, upload your part designs, and you’ll receive instant DFM feedback. The site also allows you to manage designs and orders from a single intuitive interface.
When you choose SyBridge, you’re choosing to work with a seasoned group of experts. You’ll receive the full support of our team of engineers, customer success managers, account executives, and others across the business. We’ll also leverage options for domestic and international CNC machining to ensure that your project is carried out as efficiently as possible, without sacrificing quality.